Diamond detectors pass the test of X-ray microbeam radiotherapy
A team from the LPSC, in collaboration with Inserm and the Institut Néel, has successfully tested a new X-ray microbeam controller based on diamond crystals. More resistant and faster than traditional silicon or ionisation chambers, this technology could prove decisive in the implementation of an original radiotherapy protocol, combining the 'Flash' effect and micrometric spatial fractionation of the beam, which is more effective against tumours and generates fewer side effects. The test, carried out at the Grenoble synchrotron (ESRF) as part of a veterinary clinical trial on this new protocol, validated the proper operation of the detector, which makes it possible to control the dose deposited in the patient.
The fight against cancer often requires cutting-edge technology. A collaboration between the LPSC, Inserm and the Institut Néel is proving this once again by successfully testing a detector custom-designed for use in an innovative new approach to radiotherapy: radiotherapy using intense X-ray microbeams, known as MRT (microbeam radiation therapy). This treatment, which aims to increase the effectiveness of radiotherapy while reducing its side effects, involves the administration of high doses that are extremely brief and highly localised. These are conditions for which conventional silicon detectors or gas ionisation chambers are no longer suitable: they are not fast enough, or not strong enough, or not spatially accurate enough, or not capable of accurately measuring the energy deposited in living tissue. The solution devised by scientists is a detector made of diamond. And with good reason.
Diamonds have many advantages
Diamond has many advantages. Firstly, because of its electronic properties, it can be used to manufacture detectors that generate very little background noise in normal light or temperature conditions. What's more, electrons (and their positive counterparts in semiconductors, known as holes) move much faster in diamond than in silicon, or ions in an ionisation chamber, enabling data to be taken with very high counting rates without the charge carriers recombining, and very fast signals. But that's not all: diamond is also highly resistant to radiation, because the carbon atoms are difficult to displace from their position in the crystal, even under the impact of particles or radiation. Finally, diamond has very high thermal conductivity: it easily dissipates the heat from the energy of the incoming beam.
Crystals synthesised from scratch
However, designing such a detector is not that simple. Firstly, the diamonds used here do not come from a mine. They were created in a laboratory using a special technique: vapour deposition. This method of making diamonds involves 'growing' the crystals by depositing carbon atoms on a surface from gases such as methane, which is a carbon-rich gas ionised in a plasma. This technique, which is different from that used to produce natural diamonds, makes it possible to manufacture chemically pure diamonds. However, having diamonds is not enough: they have to be included in the detector. To do this, each of the 8 diamonds cut and polished by the manufacturer was metallised on the surface at the Institut Néel in the form of micrometric tracks, using a thin conductive layer to collect the electrical charges set in motion in the diamond when the detector is operating under electrical voltage. Electronics based on printed circuits were developed at the LPSC, and placed around the diamonds to measure the flow of information from the 138 readout tracks on the crystals. It is this reading electronics, together with the exceptional qualities of the diamond, that enable this detector to monitor radiotherapy using X-ray microbeams.
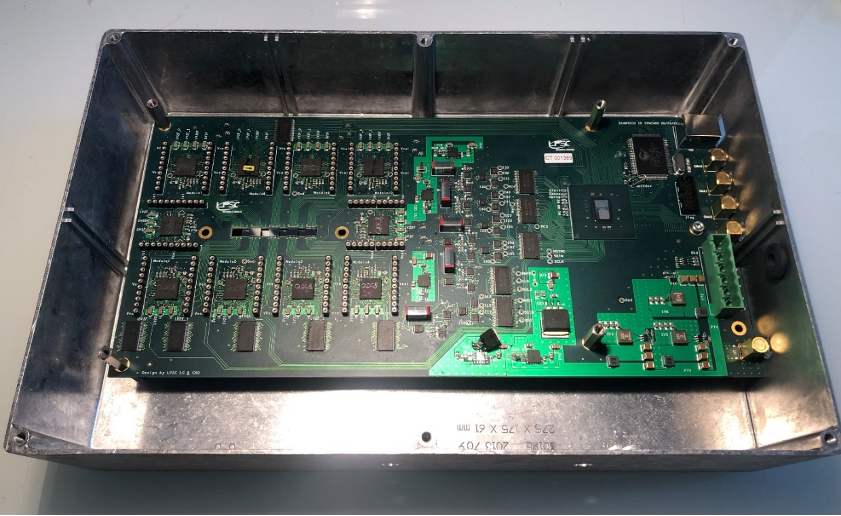
X-ray microbeam radiotherapy
The X-ray microbeam radiotherapy technique combines two effects: the so-called "Flash" effect, in which the entire dose required to destroy a tumour is delivered in a fraction of a second, and the so-called dose-volume effect: only a fraction of the tumour volume actually receives a significant dose, by scanning a comb of tiny 50-micrometre-wide beams spaced 400 micrometres apart. It is therefore based on the use of very intense X-ray microbeams. These beams deliver a high dose of radiation to a tumour over a very short period of time, destroying the cancer cells while leaving the healthy tissue to repair itself after irradiation. Cancer treatment is often based on heavy techniques that also have an impact on the healthy tissue surrounding the tumour. Radiotherapy is no exception. To limit the harmful effects, the dose of radiation delivered must be kept to a minimum, so that healthy tissue has a better capacity to repair its DNA. It is therefore vital to measure the absorbed radiation dose very precisely, and this is where the diamond detector comes in. Placed upstream and downstream of the tumour, it is capable of measuring the energy deposited very precisely, and therefore potentially of adjusting the dose delivered according to the needs of the treatment.
Undergoing tests at the Grenoble synchrotron
This detector is currently being tested at the Grenoble synchrotron (ESRF) as part of preclinical trials on dogs with brain tumours. Only one sensor is used, which measures the energy deposited after the beam has passed through the patient. Measurement of the incident flux must be carried out separately. The next step will be to manufacture a second diamond detector whose role will be to quantify the characteristics of the beam before it penetrates the tissue. This second phase will begin thanks to support from a number of sources: IN2P3 (Master-project Diamant), Grenoble IDEX (a thesis), and INCa (Cancer Plan). There will then be a detector upstream of the patient and another downstream, enabling very precise verification that the right dose has been delivered to the right place. The diamond is definitely a jewel in more ways than one!
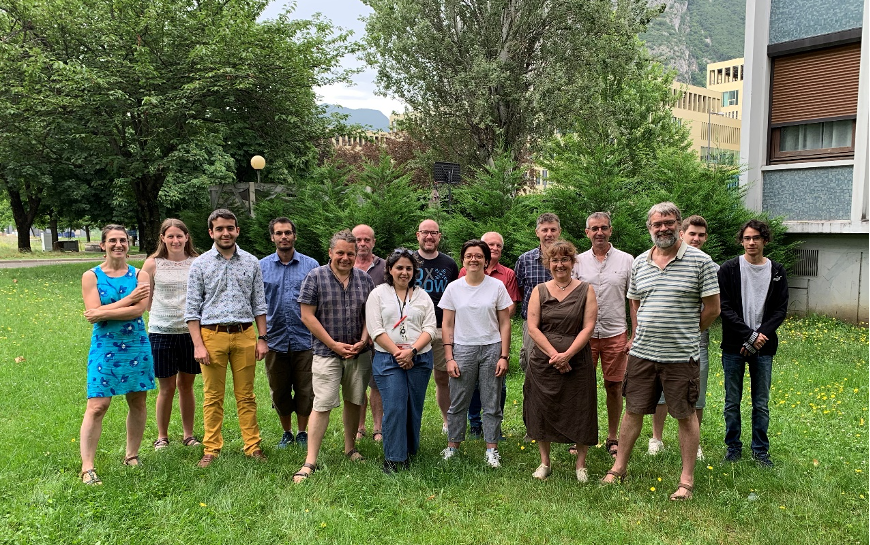
More information :
Discover two other articles (in french) on the use of diamonds:
- Des diamants pour étudier l'impact des rayonnements sur le vivant
- Du diamant pour révolutionner les détecteurs de l'IN2P3